A pool of scientists from the CNR National Research Council ISASI & IPCB has developed an original method to “package” liquids with a very thin biocompatible and biodegradable polymer membrane. Which lends itself to multiple developments for sustainable, economical and original packaging. Here is the paper published in the journal Science Advances.
Confining the water thanks to thin and biodegradable films is an attractive way to create a fast packaging that allows to control the “shape of water”. Water is one of the most important substances on Earth for our lives, it covers two thirds of the earth’s surface and is an essential means for living micro-organisms and for many technological and industrial processes.
A growing trend in the packaging industry, given the greater awareness of the importance of process sustainability, is to have biocompatible and biodegradable products. Having containers that have these characteristics and that can be easily adapted to the content, being not necessarily rigid, would represent an important step forward, especially in terms of innovation.
This article aims to illustrate the significant progress made in the packaging world, thanks to a new method that allows to coat volumes of water or aqueous-based materials with a thin film of polymer, completely enclosing and sealing the water content.
This method represents a way to create a very thin packaging around aqueous systems, coating liquids even when they take complex and transitory shapes. We could define it a packaging with reduced impact and increasingly sustainability thanks to an innovative process that respects the environment.
What is a rapid packaging?
The ability to isolate, engineer and model materials in complex two or three-dimensional shapes through bottom-up engineering techniques, on micro and macro-metric scale, is increasing importance in materials science. Today, the technicians use a variety of complex and expensive methods for micro-fabrication, including for example two-photon polymerization, interference lithography, replica molding and multistep processes for modeling and shaping the polymeric material of interest. However, most protocols require chemical and physical pre-treatments to achieve the final desired properties.
These treatments, besides being invasive with respect to the final result, are also expensive from a point of view of time and cost. However, the proposed method is a very fast process that allows to obtain a very thin film capable of coating water volumes or 3D gelatinous materials (hydrogels) in an aqueous base, and that can be activated whenever you are in the presence of a surface covered with water. The process takes place instantaneously and completely in a spontaneous way, starting from a small drop of polymer solution. The polymer used in the study is the poly (lactic-co-glycolic) acid, PLGA. The PLGA is a biodegradable and biocompatible polymer, which is dissolved in dimethylcarbonate (DMC) at a specific concentration for the packaging process. DMC is an organic solvent that easily dissolves the PLGA and evaporates slowly. When the polymeric solution (PLGA / DMC) comes into contact with a substance with a high water content (or with the same drop of water), the PLGA film is instantaneously formed around it, adapting to the geometry of the aqueous system, which acts as a guide.

When the solution comes into contact with water, the solvent is extracted and it is the variation of the surface tension that guides the instantaneous formation of the film which expands conforming to the geometry assumed by the liquid. The process takes place in a few seconds and, thanks to the slow extraction of the solvent, an homogeneous and non-porous film is generated. By controlling the amount of polymer used, it is possible to tune the thickness of the coating produced, while controlling the shape assumed by the liquid it is possible to model the silhouette of the corresponding polymeric coating.
Packaging on the water surface
As explained in the previous paragraph, when a drop of a PLGA polymer solution dissolved in DMC is placed on a water surface, the formation of a non-porous continuous film is instantaneously observed. With a bottom-up approach, we are able to cover a surface of water thanks to the self-assembling of the polymer. This process is characterized by an unprecedented versatility capable of allowing the formation of membranes able to wrap liquids or water-rich environments in different geometries. PLGA is currently widely used in the biomedical field and is part of many therapeutic devices, approved by the Food and Drug Administration (FDA), due to its biodegradability and biocompatibility. The packaging process takes place both in the case of a drop resting on a surface, and in the case of a pendant drop from an orifice or a liquid film deposited inside a container: in this case the polymer can coat and seal all the free surface of the water surface. The same process works even in case of gel volumes or in general of highly hydrated material and of any shape. The packaging method proposed is a function of the volume used, but also depends on the properties of the polymer solution (materials, viscosity, concentration). A variety of methods were used for the characterization of the membranes produced, including, for example, electronic scanning microscopy (SEM), permeability tests and characterization of mechanical properties such as Young’s modulus measurement. The acquired SEM images have revealed a non-porous symmetrical polymeric structure characterized by a homogeneous surface and thickness.
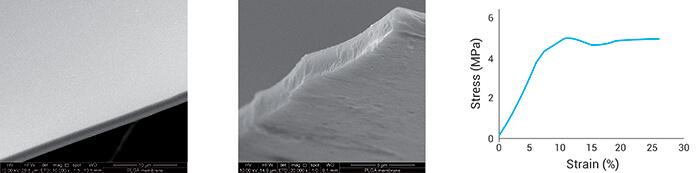
PLGA surfaces and cross sections were observed through SEM by means of a Quanta 200 FEG SEM (FEI, Eindhoven, The Netherlands) in a high-vacuum mode. Before SEM observations, membrane samples were mounted onto SEM stubs by means of carbon adhesive disks and sputter-coated with a 5- to 10-nm-thick Au-Pd layer. All the samples were observed at 10- to 20-kV acceleration voltage using a secondary electron detector. An FTA 1000 (First Ten Ångstroms, Portsmouth, VA, USA) instrument was used to measure static CAs of the PLGA membrane. The UV-visible spectrum of a PLGA membrane (thickness, about 1.5 μm) was collected on a V-570 UV spectrophotometer (Jasco, Easton, PA, USA) in the 200- to 800-nm wavelength range and with 0.5-nm resolution. Tensile tests were performed on rectangular-shaped samples (thickness, about 1.5 μm; width, 6 mm; gauge length, 10 mm) of PLGA membranes on a microtensile test apparatus (Deben, Woolpit, UK). Stress strain curves were recorded at 25° ± 1°C and 50 ± 5% relative humidity (RH) with a 200 N load cell and a crosshead speed of 0.1 mm/min. Water vapor and oxygen permeability were evaluated by means of a Multiperm apparatus (ExtraSolution, Pisa, Italy) working in a gas/ membrane/gas configuration. The exposed area of the film was 2.0 cm2. Measurements were performed at 25° ± 1°C and 50 ± 0.5% RH.
A typical stress-strain curve recorded on PLGA samples is reported in Figure 2 c. The Young’s modulus of the membrane is 75 ± 1 MPa, and the yield stress is 5.3 ± 0.2 MPa. Yielding occurs at 10 ± 2% strain, after which the sample is deformed at almost constant stress up to about 30%. These values are consistent with data reported in literature for PLGA films by considering the different thickness and test conditions. More specifically, the Young’s modulus obtained for the PLGA membranes was lower with respect to literature data because of the lower strain rate used in our experimental setup . Last, we investigated gas barrier properties of the PLGA membrane by measuring oxygen and water vapor permeability. The membrane showed high barrier properties to water vapor. Water vapor and oxygen permeability were evaluated by means of a Multiperm apparatus (ExtraSolution, Pisa, Italy) working in a gas/ membrane/gas configuration. The calculated water vapor permeability on the membrane was 140 ± 8 (g μm)/(m2 day kPa), more than one order of magnitude lower than that reported for the PLA homopolymer. Moreover, the membrane shows a very high permeability to oxygen, this parameter is very interesting for biological and biomedical applications. The calculated oxygen permeability was 1.90 × 105 ± 0.12 × 105 (cm3 μm)/ (m2 day atm), about one order of magnitude higher than the oxygen permeability of PLA. For the characterization, we focused on bulk samples consisting of flat membranes fabricated in a petri dish filled up with water. The membrane thickness, calculated by SEM observation, is 1.65 ± 0.05 μm. We performed static measurements of the water contact angle (CA) on the membrane. We found an average CA value of 73° ± 1°, typical of mildly hydrophilic polymers. Compared to conventional films of polylactic acid (PLA), the water CA of PLGA is lower because of the presence of the less hydrophobic glycolic acid segments in comparison to the lactic acid ones. The materials used in the process are: PLGA 50:50 (38,000 to 54,000 Da; PLGA Resomer RG 504H, Boeringer Ingelheim) dissolved in DMC [20 to 30 (w/v); DMC, 99%; Sigma-Aldrich)] at 25% of wheight.
The membrane formation process in the case of water-based gelatinous materials is simple and fast. The polymer / solvent solution is colored with the addition of a fluorophore to ensure a better contrast during image acquisition, see Figure 3.
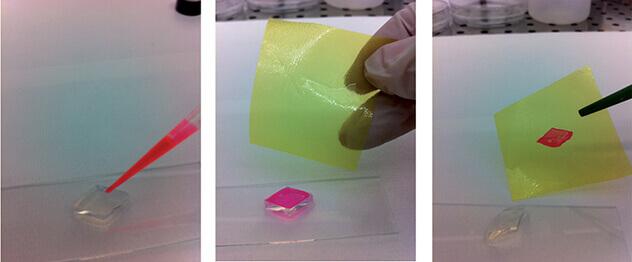
When the solution comes in contact with the free surface of the gelatinous structure, the membrane is formed instantly, adapting to the shape and the edges of the structure used as a guide. As shown in Figure 3, the rhomboidal surface appears transparent before the membrane is formed and colored at the end of the process. To demonstrate the presence of the thin polymeric film, a Teflon support is used to recover the rhomboidal membrane.
The packaging process can also be used in the case of more complex structures, such as a matrix of spheres immersed in water. In fact, following the same formation process, the spheres are completely covered by a thin polymeric film that adapts to the underlying structure and surface. In Figure 4 the reflections of the light allow to visualize the transparent packaging supported by the spheres, even at the end of the process of evaporation of the liquid in which they were immersed.
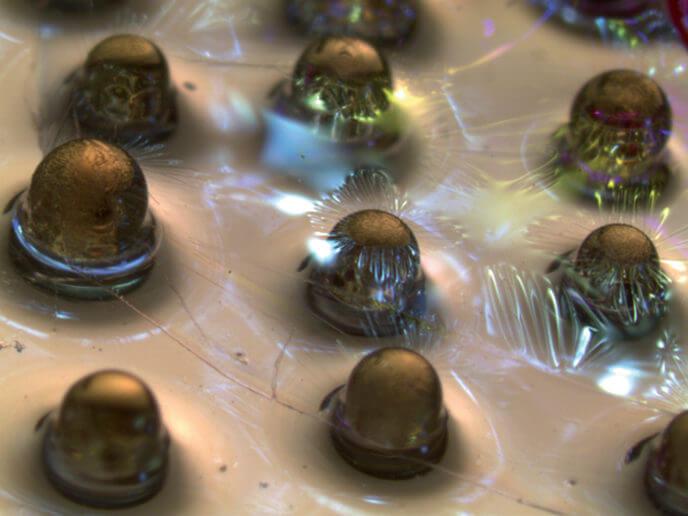
Conclusions
The method proposed represents a new engineering approach of the bottom-up technology, it is ecological and economic allowing the instant formation of a polymeric packaging in presence of water surfaces. The proposed method opens up multiple possibilities of use and applications, starting from the biomedical field, involving industrial and transformation processes, being useful for transport, preservation and protection from external agents. Furthermore, biotechnology experiments in microgravity, materials science and the study of fluid dynamics can take advantage of the possibility of creating biocompatible, biodegradable and easily modeled water containers. Further experiments and demonstrations of the results obtained on a macroscopic scale can broaden the field of application to industrial and automated approaches.